Evaluation of Vertical Patterns in Chlorophyll‐A Derived From a Data Assimilating Model of Satellite‐Based Ocean Color
Chlorophyll is found in phytoplankton, which are microscopic marine plants that capture sunlight for photosynthesis. These organisms are the foundation of the ocean’s food web, supporting everything from small fish to large marine animals like whales. During photosynthesis, phytoplankton absorb carbon dioxide (CO₂) from the atmosphere, acting as natural carbon sinks. This process helps regulate global carbon levels and contributes to mitigating climate change by lowering atmospheric CO₂. Chlorophyll concentrations are key indicators of ocean productivity and ecosystem health.
Satellite‐based sensors of ocean color have become the primary tool to infer changes in surface chlorophyll, while autonomous drifting profiling floats with biogeochemical (BGC) Argo sensors, or BGC‐Argo floats, are now filling the information gap at depth. An alternative approach for expanding surface remote sensing information to depth is the incorporation of satellite ocean color into numerical models via data assimilation (DA). An example of such application is the GMAO’s NASA Ocean Biogeochemical Model (NOBM, Figure 1), which was developed with the goal of covering gaps and correcting remote sensing retrievals using mechanistic biogeochemical and ocean circulation dynamics.
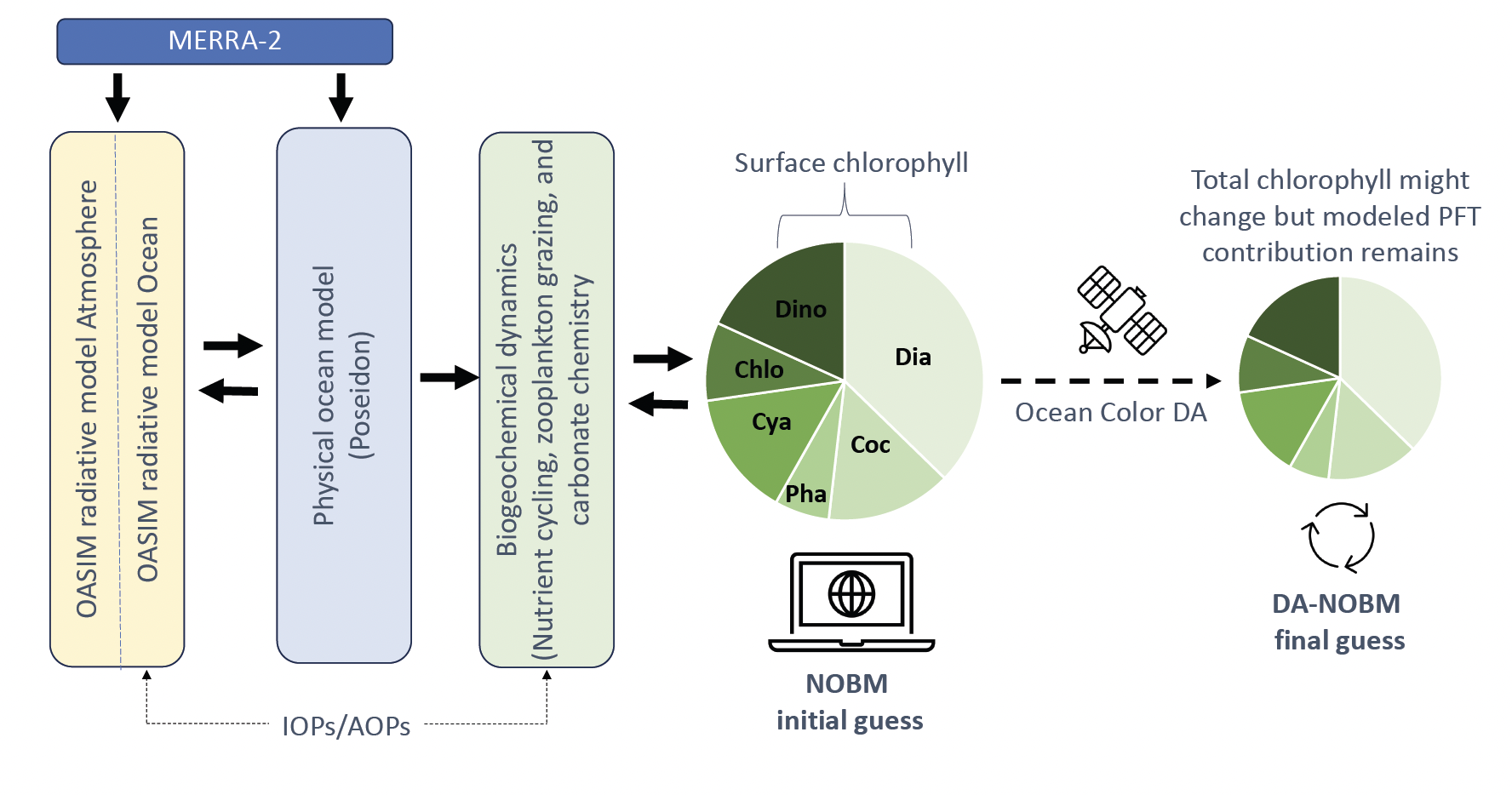
The assimilation of ocean color data into the NOBM has been shown to improve the surface representation of biogeochemical variables in the model such as chlorophyll concentration and net primary productivity. However, the vertical structure of the model output has not had been evaluated to date, mostly due to the lack of in situ biogeochemical profiles. Here, we describe the vertical structure in the NOBM's chlorophyll‐a output and compare it with BGC‐Argo float profiles from the Southern Ocean Carbon Climate and Modeling project (SOCCOM) and in situ data from the Hawaii Ocean Time‐series (HOT) program, station ALOHA (Figure 2).
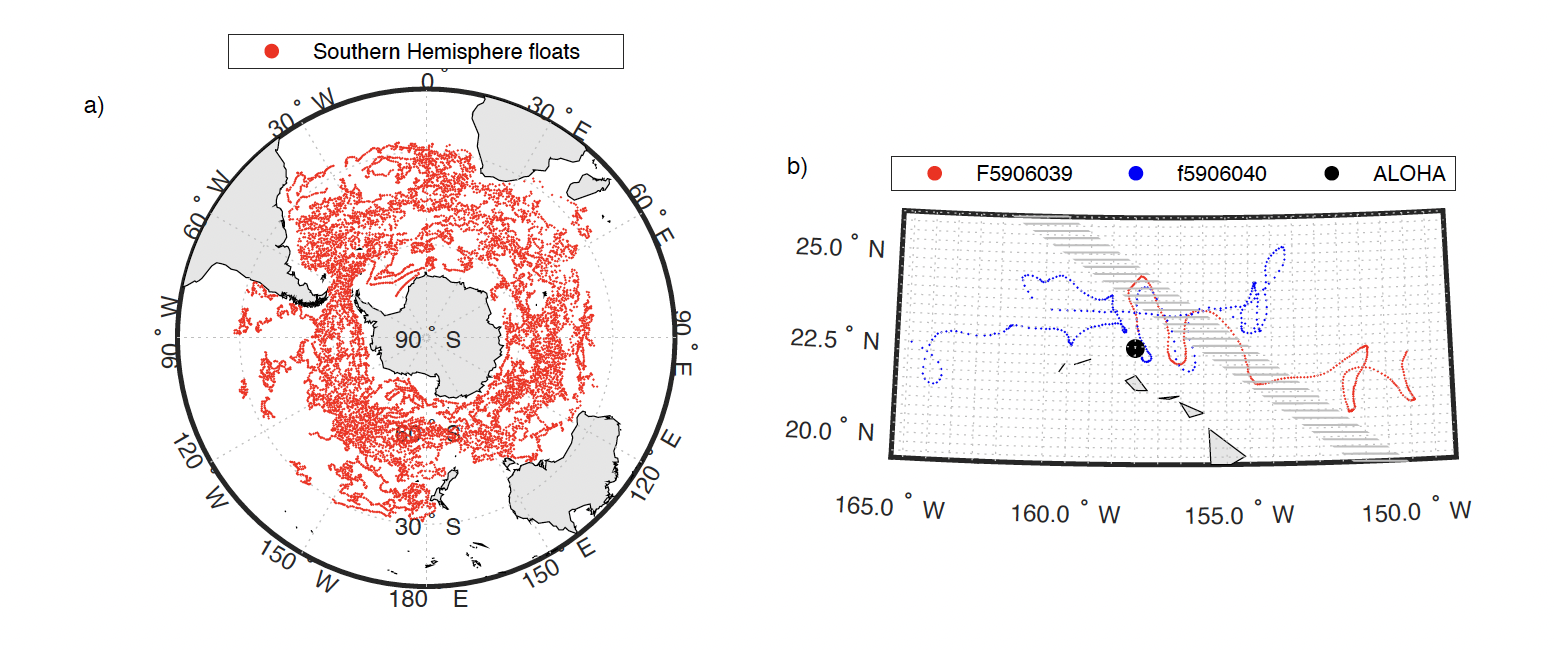
The analysis shows a generally good qualitative agreement between float and model chlorophyll output with model estimates yielding on average slightly higher chlorophyll concentration than those from the float array. The highest model Root‐Mean‐Square Error (RMSE) in the upper 50 m with respect to the float array is of 0.6 mg Chl m-3 and decreases with depth (Figure 3). This kind of accuracy should allow the detection of seasonal changes in float‐based biomass (varying between 0.01 and > 1 mg Chl m-3) but might hinder the identification of subtle changes in chlorophyll at narrow local scales.
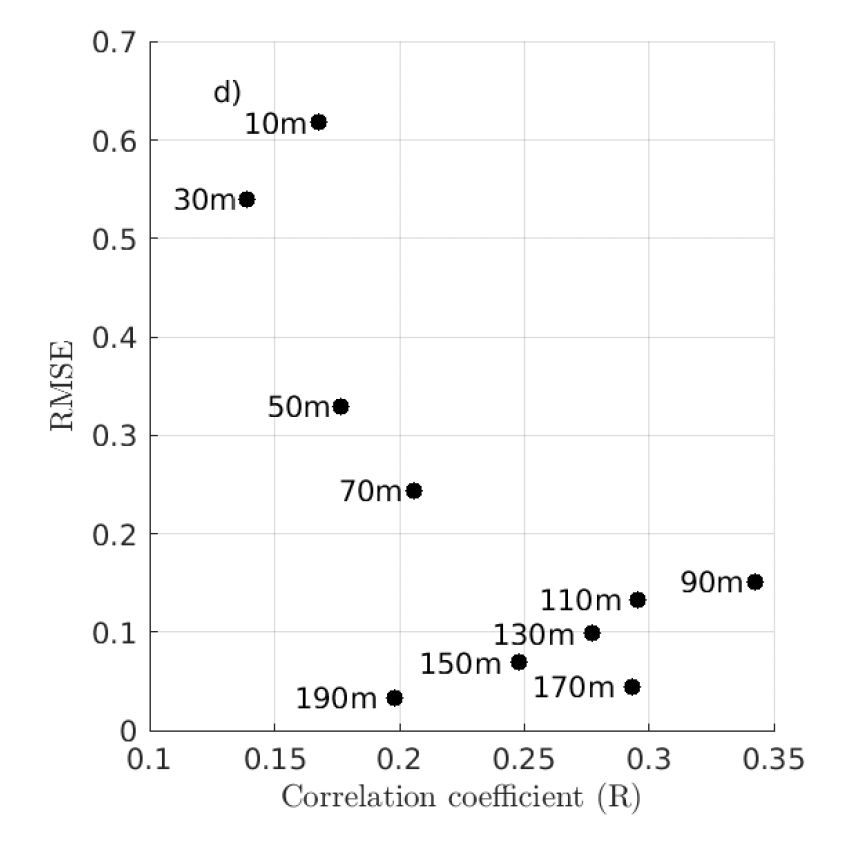
Over the Southern Ocean, region with the highest density of biogeochemical depth-profile information, the model is able to replicate the seasonality observed in depth-resolved chlorophyll-a observed in the BGC-Argo array (Figure 4). Chlorophyll seasonality is reduced in the subtropical sections of the Southern Hemisphere (north of 40S), with modeled outputs showing a clear deep chlorophyll maximum between 50 and 100 m (Figures 4b and 4d). BGC-Argo floats also present higher levels of subsurface chlorophyll with respect to the surface, but their magnitude is lower than that of the NOBM (Figures 4a and 4c). Differences between modeled and float chlorophyll indicate that the model overestimates the float array north of 40°S throughout all seasons. South of 40°S, an interesting dynamic emerges, where the NOBM overestimates float chlorophyll during the first phase of the spring bloom and underestimates it during summer and fall months. The overall mismatch between model and float data is more acute polewards and south of 50°S compared to the more subtropical sections. The NOBM is constrained by ocean color satellite observations which should minimize any temporal gap in the estimation of the seasonal “greening” of the surface ocean. However, remote sensing data in the Southern Ocean is hindered by enhanced cloudy conditions and periods of high solar zenith angle over this region (Gregg & Casey, 2007b), affecting the quality of the assimilated satellite-based ocean color data. Additionally, temporal changes in the fluorescence‐biomass relationship in phytoplankton chlorophyll not accounted by the fixed correction of float bio‐ optical profiles likely contribute to the disagreement.
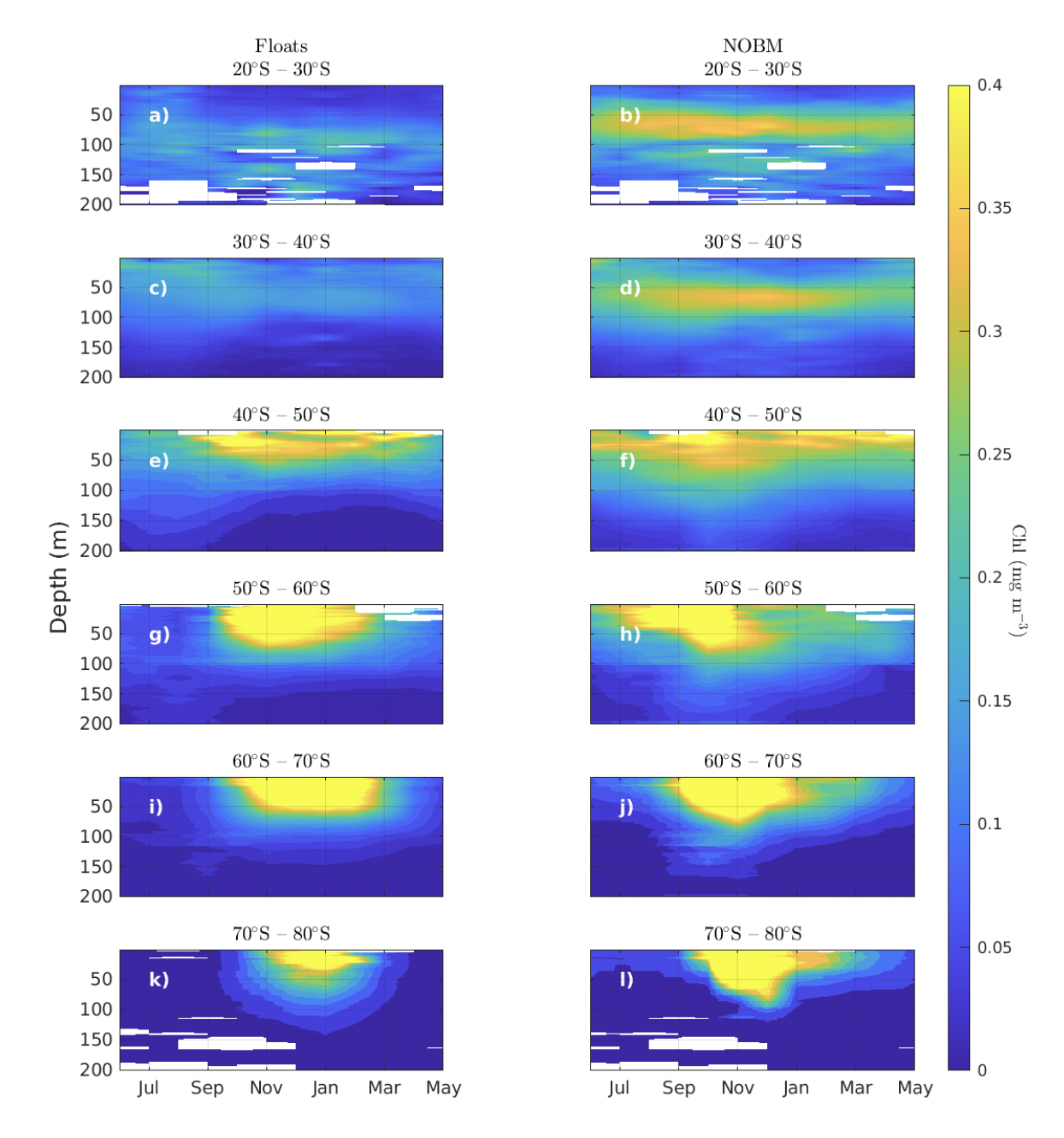
Uncertainties in chlorophyll estimates need to be quantified to use BGC‐Argo bio‐optical data as a reliable benchmark for validation purposes. However, the general qualitative agreement between data from the float array and the NOBM provides confidence in the ability of the model to replicate realistic biogeochemical features below the surface, where its output is not directly constrained by the assimilation of satellite ocean color data. On-going and future work includes updating the NOBM code to retrieve offline estimates of Chl:C ratio and conduct similar evaluations on phytoplankton carbon and associated fluxes, as well as coupling the biogeochemical model with a physical circulation model capable of resolving finer spatial scales (i.e., Modular Ocean Model version 5 and 6) as part of NASA's Goddard Earth Observing System (GEOS) models.
References:
Gregg, W. W., & Casey, N. W. (2007b). Sampling biases in MODIS and SEAWIFS ocean chlorophyll data. Remote Sensing of Environment, 111(1), 25–35. https://doi.org/10.1016/j.rse.2007.03.008